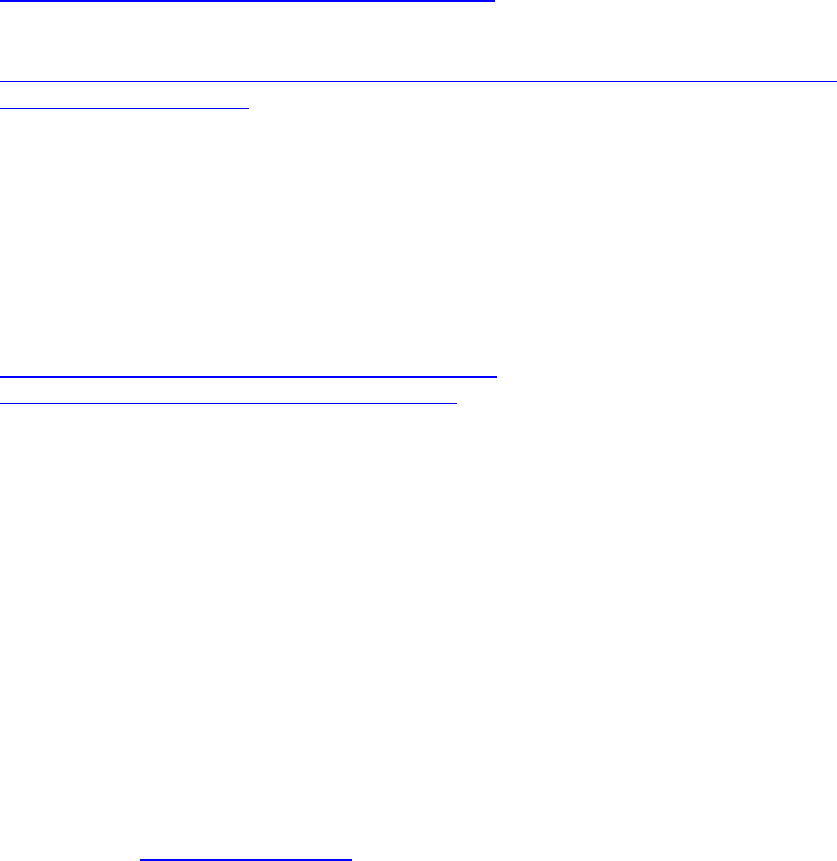
82
Greene,C.,Dimitri,C.,Lin,B.‐H.,McBride,W.,Oberholter,L.,&Smith,T.(200 9).Emergingissuesinthe
U.S.organicindustry.EconomicInformationBulletinNumber55.Retrievedfrom
http://www.ers.usda.gov/publications/eib55/eib55.pdf
Greuere,G.P.(2006).Ananalysisoftraderelatedinternationalregulationsofgeneticallymodifiedfood
andtheireffectsondevelopingcountries.Retrievedfrom
http://www.ifpri.org/publication/analysis‐trade‐related‐international ‐regulations‐genetically‐
modified‐food‐and‐their‐eff
Griffiths,B.S.,Caul,S.,Thompson,J.,Birch,A.N.E.,Cortet,J.,Andersen,M.N.,&Krogh,P.H.(2007a).
Microbialandmicrofaunalcommunitystructureincroppingsystemswithgeneticallymodified
plants.Pedobiologia,51(3),195‐206.
Griffiths,B.S.,Heckmann,L.H.,Caul,S.,Thompson,J.,Scrimgeour,C.,&
Krogh,P.H.(2007b).Varietal
effectsofeightpairedlinesoftransgenicBtmaizeandnear‐isogenicnon‐Btmaizeonsoil
microbialandnematodecommunitystructure.PlantBiotechnologyJournal,5(1),60‐68.
Hall,M.R.,Swanton,C.J.,&Anderson,G.W.(1992).TheCriticalPeriodofWeedControl
inGrainCorn
(Zeamays).WeedScience,40(3),441‐447.
Hall,R.C.,&Twidwell,E.K.(2002).Effectsofdroughtstressoncornproduction.Retrievedfrom
http://www.sdstate.edu/sdces/store/Publications/pub‐
details.cfm?customel_datapageid_858688=872114
Hart,C.E.(2006).FeedingtheEthanolBoom:WherewilltheCorncomefrom?IowaAgriculturalReview,
12,3‐5.
Haughton,A.J.,Champion,G.T.,Hawes,C.,Heard,M.S.,Brooks,D.R.,Bohan,D.A.,Clark,S.J.,Dewar,
A.M.,Firbank,L.G.,Osborne,J.L.,Perry,
J.N.,Rothery,P.,Roy,D.B.,Scott,R.J.,Woiwod,I.P.,
Birchall,C.,Skellern,M.P.,Walker,J.H.,Baker,P.,Browne,E.L.,Dewar,A.J.G.,Garner,B.H.,
Haylock,L.A.,Horne,S.L.,Mason,N.S.,Sands, R.J.N.,&Walker,M.J.(2003).
Invertebrate
responsestothemanagementofgeneticallymodifiedherbicide–tolerantandconventional
springcrops.II.Within‐fieldepigealandaerialarthropods.PhilosophicalTransactionsofthe
RoyalSocietyofLondon.SeriesB:BiologicalSciences,358(1439),1863‐1877.
Hawes,C.,Haughton,A.J.,Osborne,J.L.,Roy,D.B.,Clark,S.J.,Perry,
J.N.,Rothery,P.,Bohan,D.A.,
Brooks,D.R.,Champion,G.T.,Dewar,A.M.,Heard,M.S.,Woiwod,I.P.,Daniels,R.E.,Young,
M.W.,Parish,A.M.,Scott,R.J.,Firbank,L.G.,&Squire,G.R.(2003).Responsesofplantsand
invertebratetrophicgroupsto
contrastingherbicideregimesintheFarmScaleEvaluationsof
geneticallymodifiedherbicide–tolerantcrops.PhilosophicalTrans actionsoftheRoyalSocietyof
London.SeriesB:BiologicalSciences,358(1439),1899‐1913.
Heap,I.M.(2008).InternationalSurveyofHerbicideResistantWeeds..AccessedMarch,1,2008.
Retrievedfromwww.weedscience.com.
Henry,C.,Morgan,D.,Weekes,R.,Daniels,R.,&Boffey,C.(2003).FarmscaleevaluationsofGMcrops:
monitoringgeneflowfromGMcropstonon‐GMequivalentcropsinthevicinity(contract
referenceEPG1/5/138).PartI:ForageMaize.:DEFRA.
UtilizingDrought‐DamagedCorn,1‐4(1990).
Hoeft,R.,
Nafziger,E.,Johnson,R.,&Aldrich,S.(2000).Moderncornandsoybeanproduction.Savoy,IL:
MCSPPublications.
Holm,L.,Pancho,J.,Herberger,V.,&Plucknett,D.L.(1979).Ageographicalatlasofworldweeds.471‐
04393.
Holmén,B.,Miller,D.,Hiscox,A.,Yang,W.,Wang,J.,Sammis,T.,&Bottoms,R.
(2006).AerosolEmissions
fromFieldPlantingOperations.
Howell,T.A.,Tolk,J.A.,Schneider,A.D.,&Evett,S.R.(1998).Evapotranspiration,yield,andwateruse
efficiencyofcornhybridsdiffering inmaturity.AgronomyJournal,90(1),3‐9.